Research Article by William J. Ripple and Robert L. Beschta
College of Forestry, Oregon State University, Forest Resources, Corvallis, OR 97331, United States
published in 2006 by Elsevier in ScienceDirect.
Abstract
The strength of top-down forces in terrestrial food webs is highly debated as there are few examples illustrating the role of large mammalian carnivores in structuring biotic and abiotic systems. Based on the results of this studywe hypothesize that an increase in human visitation within Zion Canyon of Zion National Park ultimately resulted in a catastrophic regime shift through pathways involving trophic cascades and abiotic environmental changes. Increases in human visitors in Zion Canyon apparently reduced cougar (Puma concolor) densities, which subsequently led to higher mule deer (Odocoileus hemionus) densities, higher browsing intensities and reduced recruitment of riparian cottonwood trees (Populus fremontii), increased bank erosion, and reductions in both terrestrial and aquatic species abundance. These results may have broad implications with regard to our understanding of alternative ecosystem states where large carnivores have been removed or are being recovered.
Introduction
Humans can have a major role in food web dynamics by displacing or extirpating top predators. Over a half century ago, the iconoclast Aldo Leopold was among the first to argue that elimination of large mammalian predators had strong top-down influences on ecosystems (Leopold et al., 1947). Based on widespread empirical observations in the early to mid- 20th century, when large carnivores were being extirpated from significant portions of the United States, Leopold and colleagues suggested that the loss of these carnivores set the stage for ungulate irruptions and ecosystem damage. Perhaps the most notable examplewas the Kaibab plateau in Arizona (Leopold, 1943; Leopold et al., 1947; Ripple and Beschta, 2005). Widely reported in early biology and ecology textbooks as a lesson in top-down importance, the Kaibab study was more recently deleted from textbooks after alternative hypotheses for ungulate irruptions were suggested (Caughley, 1970; Burke, 1973).
Within only one to two centuries the widespread effects of Euro-American settlement and development across the continental United States resulted in range collapse for most large mammalian carnivore species (Laliberte and Ripple, 2004). Yet, potential long-term cascading effects involving the loss of these large carnivores are largely unknown.
A trophic cascade occurs when the presence of a top predator significantly affects consumers and this interaction alters or influences species composition, age structure, or spatial distribution of producers (plants). While current discussions on terrestrial food webs continue to question the relative strength of top-down forcing versus bottom-up controls (Estes, 1996; Pace et al., 1999; Terborgh et al., 1999, 2001; Polis et al., 2000; Borer et al., 2005), there is often little consideration beyond tri-trophic cascades, especially concerning potential pathways involving changes in the abiotic environment that could contribute to a loss of habitats, a loss of biodiversity, or regime shifts resulting in alternative states (Schmitz et al., 2006). Catastrophic regime shifts can occur when perturbations dramatically alter ecosystem structure and function (Holling, 1986; Scheffer and Carpenter, 2003). A regime shift typically occurs as a relatively abrupt restructuring of an ecosystem, but with prolonged consequences. The likelihood of a shift to a less desired state is increased when humans remove whole trophic levels (top-down forces), thereby reducing an ecosystem’s capacity to generate services (Folke et al., 2004).
From a theoretical perspective, a major academic debate regarding trophic cascades was initiated when Hairston, Smith, and Slobodkin proposed the Green World Hypothesis, which indicated that predators maintain global plant biomass at high levels by limiting herbivore densities (Hairston et al., 1960). Yet even with recent advances in food web ecology, the debate about the Green World Hypothesis continues due to ongoing disagreement on the frequency and relative role of top-down versus bottom-up forces, as well as a paucity of studies regarding the effects of large mammalian predators on terrestrial vegetation (Pace et al., 1999; Polis et al., 2000; Shurin et al., 2002; Borer et al., 2005).
Ecosystem properties and processes in the presence or absence of large carnivores are not widely understood. For example, there is a lack of controlled, large-scale, and long-term studies of large carnivores, prey, and plant communities. This scarcity of research is attributable to a lack of functional populations of large carnivores, the cost of such studies, the need for relatively large spatial and temporal scales, the difficulty of experimentally manipulating populations of large carnivores, and the problem of separating confounding effects including the role of humans. To date, the vast majority of trophic cascades studies in terrestrial ecosystems have taken place at the scale of meters to hectares involving very small predators over short time periods. This limits the transfer of results to large landscapes, large carnivores, and long time scales (Schmitz, 2005).
A top-down trophic cascades model would predict an increase in consumer biomass and a decrease in producer biomass following predator removal, while the bottom-up model would predict little or no change in consumer or producer biomass after their removal (Ray et al., 2005). In addition to these basic top-down linkages, many other interaction pathways resulting from predator effects are likely, such as increased species interactions, improved nutrient cycling, limited mesopredator populations, and food web support for scavengers (Soulé et al., 2003, 2005; Côté et al., 2004; Reisewitz et al., 2006). One little known pathway is a linkage from predators to consumers to producers to streambank habitats to species abundance (i.e., how the change in abiotic environment due to trophic cascades affects the occurrence of native species).
Herein we report on a discovery that appears to link the loss of predators to a catastrophic regime shift via trophic cascades (cougar → deer → cottonwoods) and changes in abiotic environmental conditions (channel morphology). We took advantage of an unplanned landscape-scale experiment to document the status of an ecosystem where the presence of a large mammalian predator (cougar) had been greatly diminished in one of two landscape areas within Zion National Park, Utah. While an experimental design that includes spatial control of “predator rare” versus “predator common” areas seldom occurs in large carnivore-trophic cascades research (Terborgh et al., 2001), such a design greatly reduces the potential for confounding interactions associated with climate, natural disturbances, or habitat. An underlying hypothesis of this study is that Fremont cottonwood tree recruitment: (1) has been low in areas where cougars are scarce and mule deer abundant (treatment, Zion Canyon); and (2) has continued to occur in areas where cougars remain common and deer scarce (control, North Creek). Additionally, in the area with a reduced cougar presence, we hypothesized that reductions in riparian trees and other palatable hydrophytic plants caused increases in bank erosion, which in turn contributed to reductions in the abundance of riparian wildflowers, amphibians, lizards, and butterflies. We considered alternative hypotheses that might affect cottonwood recruitment including climate, human interventions to stream channels, and differences in geomorphology and runoff regimes between Zion Canyon and North Creek.
History of Zion Canyon
When Zion Canyon was first settled in 1862, ranchers and homesteaders found lush vegetation in the valley bottoms and excellent grass and browse in the uplands (Dixon and Sumner, 1939). During the next five decades agricultural use of bottomlands and heavy grazing eventually caused channels to destabilize and by 1915 most homesteads had been abandoned. By 1918, when Zion National Park was established, much of the natural vegetation in the canyon had been greatly diminished and the canyon deer population was at a very low level due to intense hunting (Presnall, 1938). However, by the late 1920s vegetation recovery in the canyon was underway and deer numbers had begun to increase (Presnall, 1938). Predators also returned during this 10-year period and thus kept the deer population at a low level (Smith, 1943).
Park development accelerated during the late 1920s resulting in a dramatic increase in the annual number of park visitors, from 8400 in 1924 to 68,800 in 1934. Consequently, a reduction in the canyon’s cougar density was first noted in 1934. Zion Park Naturalist Presnall (1938) wrote “Human use of the park was, and no doubt always will be, concentrated in Zion Canyon, causing profound changes in the delicate balance between deer and their natural predators”. National Park Service biologists Dixon and Sumner (1939) similarly indicated that “The presence of these hundreds of human visitors tended to drive out the cougars, which are the chief natural enemies of the deer”. Superintendent Smith (1943) retrospectively wrote “A period of expansion in the park followed from 1924 to 1934 with the construction of highways and trails and a resulting heavy increase in visitor use which tended to frighten off the predators in the canyon due to the concentration of people”.
Study Areas
As cougars have been displaced from Zion Canyon by large numbers of human visitors since the 1930s, we used this as one of two primary study areas. Our second study area, the North Creek drainage immediately west and adjacent to Zion Canyon, is a roadless area which, like other backcountry areas of Zion National Park, has a history of supporting a stable cougar population (Nile Sorenson, Utah Division of Wildlife, personal communication, 2005). Cougar hunting is prohibited within Zion National Park but occurs outside the park. Thus, canyons within the park like those along North Creek generally serve as refugia for cougars. According to Zion National Park archival files (Zion 16051, folder 15), after the 1930s tracks and other cougar sign were found in most every section of the park outside of Zion Canyon. Currently, the park is part of a Utah state wildlife management unit encompassing nearly 4500 km2 with an estimated mule deer population of approximately 7200 (1.6 deer/km2) and an estimated cougar population of 77-110 (17-25 cougar/1000 km2; Utah Division of Wildlife files). Other predators in the Zion National Park include coyotes (Canis latrans) and a few black bears (Ursus americanus). Both of these species prey on mule deer, but mostly on newborn fawns in the spring of the year.
Present-day Zion Canyon resulted from geologic down-cutting by the North Fork of the Virgin River through Jurassic rock units of the Markagunt Plateau. Modern terraces (former floodplains) along the canyon bottom contain a variety of dry-site grasses and herbaceous plants as well as Fremont cottonwood, boxelder (Acer negundo), and ash (Fraxinus spp.) trees. Scattered patches of Baccharis (Baccharis sp.) occur within the active channel. During the 1930s the National Park Service constructed rock and wire revetments (gabions) to stabilize streambanks in portions of Zion Canyon. These efforts tended to disconnect the river from its floodplain by reducing occurrence of overbank flows. Since bare substrates are typically important sites for cottonwood regeneration (Stromberg, 1998), this altered hydrologic connectivity of a river with its floodplain thus represents a potentially significant factor affecting cottonwood recruitment. A general lack of cottonwood recruitment (i.e., growth of cottonwood seedlings into trees) over a period of many years in Zion Canyon riparian areas (Fig. 1) has caused the Park Service to investigate channel alterations as a possible approach for improving cottonwood recruitment and reversing riparian degradation (McMahon et al., 2001; Steens-Adams, 2002).
We selected North Creek as a control for our study since its watershed is adjacent to Zion Canyon (North Fork of the Virgin River) and thus has similar geology, climate, and plant communities. The elevations for our study reaches, located ~15 km apart, were both ~1300 m; watershed areas were 760 km2; and 170 km2; for Zion Canyon and North Creek, respectively. High cliffs mostly impassable to mammals separate the North Creek watershed from the North Fork of the Virgin River watershed. Human use in the North Creek study area involves limited numbers of hikers annually, whereas nearly 3 million people currently visit Zion Canyon each year.
Methods
Historical mule deer population estimates were obtained from Zion Canyon census data. These estimates were adjusted (multiplied) by a factor of 2.4, an average from two population studies [2.2 from Presnall (1938) and 2.6 from Moorehead (1976)], to account for deer present but not observed.
Within Zion Canyon, an area with high human visitation and low cougar densities since the mid-1930s, we measured the diameter of all Fremont cottonwood trees ≥1 cm in diameter at breast height (DBH) in riparian areas along three 700—900m reaches. These relatively long sample reaches were undertaken to encompass all cottonwoods within a given section of the river. The Riverwalk Reach occurs immediately upstream of the Temple of Sinawava parking lot, and has had no history of channelization or bank stabilization work. The Hereford Reach extends downstream of the parking lot and is generally free of revetments although fill from historical road construction has impinged on one streambank for a portion of the reach. Continuing downstream, the Big Bend Reach has had a history of revetment construction; however, over time the effectiveness of these structures has decreased as they have been eroded or partially buried by alluvial deposits.
In the North Creek drainage, where cougars have continued to inhabit the area, we measured the DBH of all cottonwoods (≥1 cm) along three 200m reaches, one each along the Left Fork, the Right Fork, and the main stem of North Creek. Roads and revetments are absent along study reaches in the North Fork area.
For both study areas we obtained increment cores from a subset of measured cottonwoods (≥5 cm DBH), coring individual trees 1.4m above the ground. We used this information to establish age-diameter relationships for estimating ages of uncored trees, adding 7 years to the number of rings to account for tree height growth from ground level to 1.4 m above the ground.
Measured tree diameters, in conjunction with an age- diameter relationship, were used to develop historical trends in cottonwood tree recruitment for both study areas (e.g., Beschta, 2005). Based on these trends (extending a century back in time), we quantified the strength of top-down forces in Zion Canyon in comparison to those in the North Creek drainage. To assess the strength of any potential trophic cascade, we used the natural log ratio (Borer et al., 2005) of cottonwood trees within each 10-year age class for the North Creek area (control) relative to the Zion Canyon area (treatment). The average log ratio (ū) of cottonwood frequencies was calculated by:
k
ū = ∑ [ln(yj/zj)]/k,
j=1
where yj is the average number of cottonwoods per kilometer that established in “cougars common” reaches during the jth decade, zj is average number of cottonwoods per kilometer that established in “cougars scarce” reaches during the jth decade, and k is number of decades. Decadal log ratios were averaged over two time periods: (1) before cougars became scarce (1910-1940); and (2) after cougars became scarce in Zion Canyon (1940-2000).
To further assess potential cascading effects, we undertook systematic surveys of channel dimensions, streambank condition, and hydrophytic vegetation along each study reach in late September 2005. Along the North Fork of the Virgin River in Zion Canyon, we determined wetted width, thalweg depth (deepest portion of water column), and active channel width at 10-m intervals within 400-m portions of each study reach in early September 2005, using these to calculate a wetted width/thalweg depth ratio for the reach.
We took the same type of measurements in the North Creek drainage, but since these streams were smaller, the interval between measurements was 5 m along each 200-m study reach. During reach surveys, the presence of an eroding bank and the dominant hydrophytic vegetation was determined at the genus level [rushes (Juncus spp.), cattails (Typha sp.), scouring rush (Equisetum sp.)] within a 0.25m2area of streambank at the edge of the channel. Both were recorded at 5-m intervals for each side of the channel.
To assess biodiversity, we determined the relative abundance of selected indicator species, using visual encounter surveys conducted along the bank on each side of the channel. All indicator species were easily observable and occurred in both study areas. We undertook these surveys on clear sunny days during the 16-20 September 2005, between 1000 and 1700 h. Species counts involved scanning the ground surface within 2-m wide transects along the streambanks of the study reaches (Heyer et al., 1994). Surveys were completed for cardinal flower (Lobelia cardinalis), Welsh aster (Aster welshii), red spotted toad (Bufo punctatus), canyon tree frog (Hyla arenicola), and all lizard species. To determine the relative abundance of deer, we recorded each hoofprint within these belt transects. Butterflies encountered within 10-m wide belt transects along the banks of each study reach were recorded at the subfamily level. Close focusing binoculars were used to help identify lizard species and butterfly subfamilies. To index the relative abundance of cougar, we conducted a survey of fecal droppings (scat) along ∼4000m of extant foot trails in both North Creek and Zion Canyon study areas, quantifying cougar droppings as number of droppings per linear kilometer of trail.
Results
With increasing numbers of park visitors and decreasing numbers of cougars throughout the 1930s, the Zion Canyon deer population irrupted from <80 in 1930 (<4 deer/km2) to a peak of ∼600 (30 deer/km2) in 1942 (Fig. 2, Table 1). Because of impacts to vegetation, park officials trapped and removed 116 deer in 1938 (Dixon and Sumner, 1939) and shot and killed 180 more deer in 1943 (Fagergren, 1943). Between 1938 and 1947, 780 deer were killed or removed from Zion Canyon by the Park Service (National Park Service Archives, Zion 16051, folder 15). Since the 1940s canyon deer populations have declined to a recent estimate of ∼200 animals (∼10 deer/km2), which is greater than the pre-1930 levels (Fig. 2) as well as current mule deer densities outside the park (1.6 deer/km2).
Estimated number of mule deer in Zion Canyon during the 20th century. Cougars became scarce in Zion canyon by the mid-1930s, a period of dramatic increase in park visitation. The shaded band represents an estimate of uncertainty.
Sources: Mule deer data from Presnall (1938), Dixon and Sumner (1939), and the following reports located in the Zion National Park archives — 1930 Zion Park Superintendent report; 1943 chief ranger report by F.C. Fagergren; anonymous memorandum on resurgence of pink eye, 21 December 1993; National Park Service Archives, Zion 16051, folders 15 and 16.
Date | Comment |
---|---|
1932 | “The deer within the park are increasing in spite of hunting outside…” |
1933 | “Cougar signs were quite numerous within Zion Canyon this year.” |
1934 | “Evidence of cougars within the park is more readily observed, indicating perhaps they are somewhat on the increase. However, they do not come into the valley of Zion Canyon, which is one of the factors assisting in the increase of the deer population here.” |
1935 | “Deer are in the best of condition, and forage is plentiful, because of the large amount of moisture received during the winter and spring.” |
1936 | “Evidence of [deer] overpopulation in the valley of Zion Canyon, however, continues to be seen… there are about 125 deer that winter here… the number of cougar within the park is increasing [outside of Zion Canyon].” |
1937 | “The over-population of deer in Zion Canyon, which was reaching the stage where plans for reduction were under discussion, was somewhat relieved by the severe winter of 1936-1937, which some of the less healthy animals were unable to survive.” |
1938 | “The deer in Zion [canyon] are in very poor flesh and present a sorry spectacle. Professional wildlife visitors to the park this summer have been unfavorably impressed by the unbalanced deer forage situation here.” |
1939 | “Deer became too numerous on the valley floor for the amount of forage available, so 130 of these animals were trapped and transported to outside areas.” |
1940 | “The trapping and shipping of surplus Rocky Mountain mule deer from the floor of Zion Canon was carried on… 62 animals were removed from the park this year.” |
1941 | [Report not available] |
1942 | “Overpopulation of deer in Zion Canyon, with consequent scarcity of feed, is one of the major problems at present in Zion National Park. During the winter of 1941-1942, 52 deer were trapped and removed… Vegetation is so over-browsed that it is in serious condition and there is danger of complete destruction.” |
The number of tree rings (t) was highly correlated with DBH (x) for cottonwoods in Zion National Park (r2 = 0.75, n = 51, t = -0.0096x2 + 2.09x). Based on estimated cottonwood ages from DBH measurements in the sampled reaches, two age structures are apparent when comparing the North Creek study reaches with those of Zion Canyon. The North Creek area (Fig. 3a) shows continuous recruitment over time with more young trees than old ones — a normal feature of functioning gallery forests (Beschta, 2005).
In contrast, study reaches in Zion Canyon (Fig. 3b) show a general paucity of young trees and a lack of recruitment since the 1930s as an apparent result of heavy browsing pressure since then. As an index of the relative strength of this potential trophic cascade, the average log ratio of cottonwood frequencies for the North Creek/Zion Canyon areas was near zero (-0.25) for the decades before cougars became scarce in Zion National Park and relatively high (3.85) afterward. While some young cottonwoods had established in Zion Canyon during the 1970s and 1980s (Fig. 3b), nearly all were observed growing in refugia sites inaccessible to deer, such as the base of steep slopes along the river (Fig. 4a) or within the protection of Baccharis (a relatively unpalatable shrub) thickets (Fig. 4b).
As suggested by food-web theory, Fig. 5a shows an alternating pattern of biomass levels across trophic levels. In the North Creek area (control), human and consumer levels show low abundance while predator and producer trophic levels show high abundance. In Zion Canyon (treatment), the opposite pattern occurs. Although streams throughout Zion National Park experienced high flows (10—20 year return period) in January 2005, the occurrence of eroding banks for study reaches in Zion Canyon were >2.5 times as frequent as those in the North Creek area (Fig. 5a). Similarly, width/depth ratios for reaches in Zion Canyon are approximately double those in the North Creek area. For the Zion Canyon reaches active channel widths (x = 34.2 m, standard error [SE] = 1.4 m) were more than double the wetted widths (x = 15.9m, SE = 0.4 m) as a result of bank erosion into historical floodplains, which created large areas of exposed gravels along these reaches.
The relative abundance of hydrophytic plants, wildflowers, amphibians, lizards, and butterflies observed along streams in the North Creek area was higher than in Zion Canyon (Fig. 5b). Neither of the wildflower species were observed within the belt transects in Zion Canyon, but both were found growing outside the belt transects in nearby side canyon areas, physically protected from deer browsing. Lizard species observed in both “cougars common” and “cougars rare” areas include: Eastern Fence Lizard (Sceloporus undulatus), Sagebrush Lizard (Sceloporus graciosus), and Plateau Whiptail (Cnemidophorus velox). Additional lizard species observed in the “cougars common” area include: Desert Spiny Lizard (Sceloporus magister), Common Side-Blotched Lizard (Uta stansburiana), and Tree Lizard (Uta ornata). Butterfly subfamilies observed in both the “cougars common” and “cougars rare” areas include (scientific names listed as family: subfamily): Whites (Pieridae: Pierinae), True Brush-Foots (Nymphalidae: Nymphalinae), Admirals (Nymphalidae: Limenitidinae), and Grass Skippers (Hesperiidae: Hesperiinae). Additional butterfly subfamilies observed only in the cougars common area include: Swallowtails (Papilionidae: Papilioninae), Sulphurs (Pieridae: Coliadinae), Blues (Lycaenidae: Polyommatinae), Satyrs (Nymphalidae: Satyrinae), Monarchs (Nymphalidae: Danainae), and Spread-wing Skippers (Hesperiidae: Pyrginae).
Discussion
Our data confirm a major gap in the cottonwood age structure in Zion Canyon (cougars rare) occurred after 1940, where we found, on average, only 23 post-1940 cottonwoods per kilometer of stream (Fig. 3). This compares to 892 post-1940 cottonwoods per kilometer in the North Creek study reaches (cougars common). These results are consistent with a trophic cascade initiated regime shift in that the reduction in a large predator may have produced important ecosystem changes, including greater herbivore densities, decreased tree frequencies, more bank erosion, altered channel dimensions, and decreased riparian biodiversity (Fig. 6).
The geomorphic transformation of stream channels due to accelerated bank erosion can have serious ecological consequences (National Research Council, 2002). For example, plant loss on streambanks decreases shading on the surface of the water and allows width/depth to increase, thus contributing to high summertime water temperatures. Increased streambank erosion not only removes formerly deposited alluvium that serves as substrate for riparian vegetation, but reduces the capability of the stream to maintain hydrologic connectivity with its historical floodplain (Beschta and Ripple, 2006). Bank erosion also truncates allochthonous inputs from streamside vegetation, an important source of organic carbon for many aquatic organisms. An influx of finer sediments from accelerated bank erosion can decrease the median substrate size and sorting of sedimentary material in the bed, making it less inhabitable for many invertebrates that live within the interstices of the coarse bed materials (Smith, 2004). While large portions of the channel in Zion Canyon now have bare substrates normally favorable for cottonwood seedling establishment (Fig. 6b), it appears that annual herbivory since 1940 has effectively truncated the capability of seedlings to grow above the browse level of deer.
Not only do hydrophytic plants have a key role along channel margins in helping to maintain stable banks and performing other important functions, but they also provide food-web support and protective cover for amphibians, reptiles, and aquatic species. Both wildflower species assessed in this study grow in wet meadows and on moist streambanks and serve as host plants for several butterfly species. Relative low numbers of frogs, toads, and lizards associated with the “cougars rare” sites may be a result of both lower levels of invertebrate prey abundance, as well as degraded habitats with less cover for thermal regulation and protection against predators. Butterfly differences may be due to reduced diversity and abundance of flowering plants in Zion Canyon relative to the North Creek area. It should be noted that the results from our survey of amphibians, lizards and butterflies within the belt transects represent what we observed over a limited period of time and should not be considered an exhaustive inventory of these species groups. We also observed a wide range of woody browse species such as willow (Salix spp.), Squawbush (Rhus trilobata), and others in the North Creek drainage, which were absent or rare on comparable sites in Zion Canyon.
Our results are consistent with previous studies regarding the role of wolves (Canis lupus) in trophic cascades (McLaren and Peterson, 1994; Ripple and Larsen, 2000; Ripple and Beschta, 2004; Beschta, 2005; Hebblewhite et al., 2005). These studies and others (Rooney and Waller, 2003) indicate that ecosystems can be profoundly altered by ungulates after large carnivores are removed. Recent research has also connected wolves to stream channel and floodplain characteristics. For example, heavy elk browsing of willow communities after the loss of wolves ultimately generated major changes in floodplain functions and channel morphology (Beschta and Ripple, 2006). Other researchers have documented a reduction in abundance and diversity of birds in areas without wolves and with abundant ungulate populations (McShea and Rappole, 2000; Berger et al., 2001). Like wolves, cougars are a wide-ranging predator whose geographic distribution and predation effects have been greatly reduced by humans. In contrast to wolves, cougars are solitary predators.
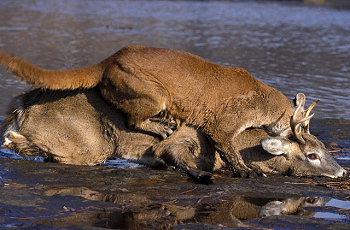
Mule deer represents the primary prey of cougars in southern Utah and are common across much of the region. However, cougars generally have avoided areas with high levels of human activity (Van Dyke et al., 1986), such as Zion Canyon. Thus Zion Canyon functions as refugia for mule deer from cougar predation due to high human presence, while areas such as the North Creek drainage provide refugia for cougars due to low human presence. These landscape-scale refugia are consistent with behaviorally mediated trophic cascades theory involving predation risk effects (Ripple and Beschta, 2004; Hebblewhite et al., 2005).
The natural log ratio of 3.85 (indicating a 47-fold difference) for young cottonwood frequencies in our control area (North Creek drainage) versus treatment area (Zion Canyon) ranks high compared to a recent meta-analysis of 114 trophic cascades studies from both aquatic and terrestrial systems, where such log ratios ranged from -0.7 to 3.2 (Borer et al., 2005). Thus, the diminishment of gallery cottonwood forests (and perhaps a wide range of other palatable species) reflects a potentially strong trophic cascade effect relative to other studies. Additionally, our results document an apparent catastrophic regime shift using a pathway spanning from trophic cascades to impacts on the abiotic environment, and ultimately, to a loss of native species abundance. Thus, the sustainability of functional riparian habitats and biodiversity may require the long-term effects of predation on herbivores, including lethal effects (densities) as well as nonlethal effects (predation risk).
Our findings on trophic cascades in Zion National Park are consistent with those of over a half century ago by Aldo Leopold regarding the Kaibab Plateau, located less than 100 km to the southeast. Zion serves as a replicate to the classic Kaibab study, since cougar/mule deer are the primary predator/prey combination in both areas. In addition, recent analyses of aspen tree rings from the Kaibab (Binkley et al., 2005) are consistent with Leopold’s hypothesis of extreme deer herbivory following predator removal, as well as the importance of predation in controlling deer populations. Aspen recruitment on the Kaibab largely terminated following predator reductions, similar to the patterns of cottonwood recruitment in Zion Canyon after cougars became scarce.
It is interesting to note that increased cougar sightings have occurred in Zion Canyon in recent years. This may be attributable to the dramatic decrease in vehicle traffic since a bus system was implemented in 2000. Reduced vehicle traffic may have positive effects on long-term riparian/aquatic biodiversity if cougars have an increasing presence in Zion Canyon. However, we are unsure how rapidly or to what extent any increase in cougar numbers in Zion Canyon might be able to reverse decades of direct impacts by deer upon riparian plant communities and subsequent impacts to the stream system.
While our results are consistent with trophic cascade theory, we nevertheless considered alternative scenarios that might affect cottonwood recruitment: (1) fluctuations in climate; (2) human interventions to channels (e.g., revetments in Zion Canyon); and (3) site attributes associated with differences in geomorphology or runoff regimes between Zion Canyon and North Creek. Both Zion Canyon and North Creek study areas had comparable cottonwood recruitment during the pre-1940 period (Fig. 3), indicating they were well-paired for comparative purposes. Thus, it would appear unlikely that any shifts in climate during the 20th century have been a significant factor associated with the post-1940 cessation of cottonwood recruitment in Zion Canyon, as a similar pattern was not observed in North Creek.
We considered the potential role of revetments constructed in the early 1930s along portions of the North Fork of the Virgin River in Zion Canyon. Such structures can diminish cottonwood recruitment by locally reducing: (1) availability of bare substrates (important for cottonwood germination); and (2) occurrence of overbank flows. Since construction of these structures decades ago, their capability to function has slowly deteriorated as they have been increasingly eroded or buried by alluvium during periods of high flow. Furthermore, our results indicated relatively low cottonwood recruitment since 1940, even in those reaches of Zion Canyon where revetments had not been constructed. Thus, some mechanism other than revetment construction appears to have been causing the post-1940 lack of cottonwood recruitment. This is further supported by observations in Zion Canyon which indicate that cottonwood recruitment is occurring, but only in localized places generally inaccessible to deer (e.g., steep canyon toeslopes, Baccharis thickets, Fig. 4).We generally observed extremely high levels of deer browsing on cottonwood seedlings in Zion Canyon, but very low levels of browsing on seedlings in the North Creek area.
Drainage areas and geomorphic features associated with Zion Canyon and the North Creek areas represent essentially constant conditions over our study period and thus are an unlikely candidate for causing a differential effect on cottonwood recruitment over time. However, to explore this issue further we measured cottonwood trees, channel width/depth ratios, and percent eroding banks along a 400-m reach of the East Fork of the Virgin River. Land use history in the East Fork during the late 1800s and early 1900s was similar to that which occurred in Zion Canyon. However, since inclusion into the Zion National Park in 1918, the East Fork has not had any roads or revetments, has experienced only a few human visitors annually, and has had continued presence of cougars. Although the East Fork has a drainage area approximately the same as that of Zion Canyon, annual peak flows average approximately 30% lower.
Along the East Fork we found 1216 young cottonwoods per kilometer (originating post-1940), with an exponential ageclass distribution very much like that of study reaches in the North Creek drainage (i.e., Fig. 3a). The frequency of eroding banks averaged only 2% for the East Fork relative to 18.9% (SE = 1.3%) for study reaches in the North Creek drainage. Both were well less than the 51.0% (SE = 3.6%) eroding banks measured in Zion Canyon. In addition, the average wetted width/depth ratio of 12.9 m/m for the East Fork was similar to the North Creek study reaches (x = 15.6m/m, SE = 4.7 m/m), but considerably less than that for Zion Canyon (x = 31.1m/m, SE = 1.6). These findings support our contention that pre-park land use history as well as stream/watershed size did not cause the post-1940 lack of cottonwood recruitment in Zion Canyon.
We postulated that the apparent regime shift in riparian plant communities and channel morphology may have influenced native fish species abundance in the North Fork of the Virgin River. Therefore, we summarized recent fish surveys for North Creek, as well as for both the North and East Forks of the Virgin River. Annual monitoring of these streams has been ongoing since 1994 (n = 11 years) and is accomplished at one fixed area station per stream using electro-shocking methods (Morvilius et al., 2006). Summaries of these annual data show mean densities of native fish over three times higher in streams within areas with cougars common (North Creek and East Fork of the Virgin River) relative to the area with cougars rare (North Fork of the Virgin River, Fig. 7). Virgin spindace (Lepidomeda mollispinis), one of four native species found in these surveys, has been proposed for federal endangered species listing because of declining populations due to habitat degradation (Morvilius et al., 2006). These results provide additional evidence of potential connectivity between terrestrial and aquatic processes linked by a trophic cascade across ecosystems, a process rarely identified in the ecological literature (Knight et al., 2005).
We hypothesize that the lack of cottonwood recruitment associated with riparian areas in Zion Canyon indicates an altered trophic cascade involving decades of low cougar densities. Subsequent impacts to the riparian/aquatic systems appear to have included reduced bank vegetation, increased bank erosion and width/depth ratios, and decreased riparian biodiversity. Thus, removing or maintaining a large carnivore appears to have had profound effects on lower trophic levels, as well as multiple indicators of ecosystem status and native species abundance. Unless changes occur at the top of the food chain, Fremont cottonwoods in Zion Canyon may ultimately disappear. While loss of cottonwoods alone represents a major impact to biodiversity, it likely chronicles other functional losses already incurred by the larger community of riparian plants and animals. In contrast, where access by humans has been restricted and cougar populations have remained intact, as in the North Creek area, browsing levels have remained relatively low, as indicated by long-term patterns of cottonwood recruitment. Furthermore, streambank erosion was low, channels relatively narrow and deep, and relative abundances of hydrophytic plants and other indicator species of biodiversity were high in this area.
Understanding the historical context of various factors affecting Zion Canyon and North Creek ecosystems is fundamental to interpreting present-day ecosystem structure and functions. This indicates not only an important need to understand how ecosystems affected by humans currently function in comparison to relatively unaffected ecosystems, but that historical patterns of human disturbances and alterations leading up to the present need be understood so that on-going effects can be realistically evaluated. With the assistance of cottonwood age—diameter relationships and stand measurements of tree diameters, we were able to reach back over a century to help assess temporal stand structural dynamics of the gallery forests within our study sites. However, without the availability of control reaches (where cougar populations remained intact over time), we would have been unable to decipher the potential effects of predator removal on abiotic indicators of ecosystem conditions and on other biotic indicators with life cycles much shorter than cottonwoods.
Our results identify a central issue for ecological studies, since the presence or absence of a top predator may have a major influence on the outcomes of such studies. Following the loss of top-down control, succession may proceed via abrupt regime shifts and alternative states (Folke et al., 2004; Schmitz et al., 2006). Without an appreciation of this context, future studies, as well as many currently in the published literature, are likely to provide conflicting results regarding function and structure of perturbed ecosystems. This may be true not only for those in southern Utah, but also across many ecosystems in the United States and around the world, where key predators (large mammalian carnivores) have been removed and consumers (e.g., increased wild ungulate populations; high densities of domestic ungulates) have significantly affected native species biodiversity.
Acknowledgements
The authors thank Cristina Eisenberg, Sally Hacker, Brian Miller, Deanna Olson, Dan Rosenberg, and Oswald Schmitz for helpful comments on an early draft of this paper; additional comments and suggestions were provided by three anonymous reviewers. Funding was provided by the National Park Service, Cooperative Agreement CA# H1200040002. Dave Sharrow and Denise Louise of Zion National Park provided technical assistance on this project. Richard Fridell and Megan Morvilius of the Utah Division of Wildlife Resources provided the fish data used in this manuscript.
References
Berger, J., Stacey, P.B., Bellis, L., Johnson, M.P., 2001. A mammalian predator-prey imbalance: grizzly bear and wolf extinction affect avian neotropical migrants. Ecological Applications 11, 967—980.
Beschta, R.L., 2005. Reduced cottonwood recruitment following extirpation of wolves in Yellowstone’s northern range. Ecology 86, 391—403.
Beschta, R.L., Ripple,W.J., 2006. River channel dynamics following extirpation of wolves in northwestern Yellowstone National Park. Earth Surface Processes and Landforms, doi:10.1002/esp. 1362.
Binkley, D., Moore, M.M., Romme, W.H., Brown, P.M., 2005. Was Aldo Leopold right about the Kaibab deer herd? Ecosystems 9, 1—16.
Borer, E.T., Seabloom, J.B., Shurnin, K.E., Anderson, C.A., Blanchetter, B., Halpern, B.S., 2005. What determines the strength of a trophic cascades? Ecology 86, 528—537.
Burke, C.J., 1973. The Kaibab deer incident: a long-persisting myth. BioScience 23, 113—114. Caughley, G., 1970. Eruption of ungulate populations, with emphasis on Himalayan tahr in New Zealand. Ecology 51, 53—72.
Côté, S.D., Rooney, T.P., Tremblay, J., Dussault, C., Waller, D.M., 2004. Ecological impacts of deer overabundance. Annual Review of Ecology, Evolution, and Systematics 35, 113—147.
Dixon, J.S., Sumner, E.L., 1939. The deer problem, deer trapping, and deer removal at Zion Canyon, Utah. In: Transactions of the 4th North American Wildlife Conference, February 13—15, 1939, Detroit, MI, pp. 231—236.
Estes, J.A., 1996. Predators and ecosystem management. Wildlife Society Bulletin 24, 390—396.
Fagergren, F.C., 1943. Memorandum for the Superintendent, report of deer reductions December 17, 1943, Zion National Park Archives, 4 p.
Folke, C., Carpenter, S., Walker, B., Scheffer, M., Elmqvist, T., Gunderson, L., Holling, C.S., 2004. Regime shifts, resilience, and biodiversity in ecosystem management. Annual Review of Ecology Evolution and Systematics 35, 557—581.
Hairston, N.G., Smith, F.E., Slobodkin, L.B., 1960. Community structure, population control, and competition. American Naturalist 94, 421—425.
Hebblewhite, M., White, C.A., Nietvelt, C.G., McKenzie, J.A., Hurd, T.E., Fryxell, J.M., Bayley, S.E., Paquet, P.C., 2005. Human activity mediates a trophic cascade caused by wolves. Ecology 86, 2135—2144.
Heyer, W.R., Donnelly, M.A., McDiarmid, R.W., Hayek, L.C., Foster, M.S. (Eds.), 1994. Measuring and Monitoring Biological Diversity: Standard Methods for Amphibians. Smithsonian Institution Press, Washington, DC, p. 64.
Holling, C.S., 1986. The resilience of terrestrial ecosystems: local surprise and global change. In: Clark, W.C., Minn, R.E. (Eds.), Sustainable Development of the Biosphere. Cambridge University Press, Cambridge, pp. 292—317.
Knight, T.M., McCoy, M.W., Chase, J.M., McCoy, K.A., Holt, R.D., 2005. Trophic cascades across ecosystems. Nature 437, 880—883.
Laliberte, A.S., Ripple, W.J., 2004. Range contractions of North American carnivores and ungulates. BioScience 54, 123—138.
Leopold, A., 1943. Deer irruptions. Wisconsin Academy of Sciences, Arts, and Letters 35, 351—366.
Leopold, A., Sowls, L.K., Spencer, D.L., 1947. A survey of overpopulated deer ranges in the United States. Journal of Wildlife Management 11, 162—183.
McLaren, B.E., Peterson, R.O., 1994. Wolves, moose and tree rings on Isle Royale. Science 266, 1555—1558.
McMahon, J.P., Moody, T., Apfelbaum, S.I., Lehnhardt, S.M., LaGesse, V., 2001. The potential for restoration along the Virgin River in Zion National Park. Grand Canyon Trust, St. George, Utah, Final Report, 57 p.
McShea, W.J., Rappole, J.H., 2000. Managing the abundance and diversity of breeding bird populations through manipulation of deer populations. Conservation Biology 14, 1161—1170.
Moorehead, B.B., 1976. Seasonal movements and abundance of mule deer (Odocoileus hemionus hemionus), in Zion National Park, Utah. Unpublished report, Utah State University, 56 p.
Morvilius, M.K., Fridell, R.A., Huebner, J.A., 2006. Virgin Spinedace (Lepidomeda mollispinis mollispinis) Population Monitoring Summary 1994—2005. Publication number 06-01, Utah Division of Wildlife Resources, Salt Lake City, Utah.
National Research Council, 2002. Riparian Areas: Functions and Strategies for Management. National Academy of Science, Washington, DC.
Pace, M.L., Cole, J.J., Carpenter, S.R., Kitchell, J.F., 1999. Trophic cascades revealed in diverse ecosystems. Trends in Ecology and Evolution 14, 483—488.
Polis, G.A., Sears, L.W., Huxel, D.R., Strong, J.M., 2000. When is a trophic cascades a trophic cascade? Trends in Ecology and Evolution 15, 473—475.
Presnall, C.C., 1938. A survey of the deer situation in Zion Canyon, Utah. Proceedings of the Utah Academy of Sciences, Arts, and Letters 15, 107—110.
Ray, J.C., Redford, K.H., Steneck, R.S., Berger, J. (Eds.), 2005. Large Carnivores and the Conservation of Biodiversity. Island Press, Washington, DC.
Reisewitz, S.E., Estes, J.A., Simenstad, C.A., 2006. Indirect food web interactions: sea otters and kelp forest fishes in the Aleutian archipelago. Oecologia 146, 623—631.
Ripple, W.J., Beschta, R.L., 2004. Wolves and the ecology of fear: can predation risk structure ecosystems? BioScience 54, 755—766.
Ripple, W.J., Beschta, R.L., 2005. Linking wolves and plants: Aldo Leopold on Trophic Cascades. BioScience 55, 613—621.
Ripple,W.J., Larsen, E.J., 2000. Historic aspen recruitment, elk, and wolves in northern Yellowstone National Park, USA. Biological Conservation 95, 361—370.
Rooney, T.P., Waller, D.M., 2003. Direct and indirect effects of white-tailed deer in forest ecosystems. Forest Ecology and Management 181, 165—176.
Scheffer, M., Carpenter, S.R., 2003. Catastrophic regime shifts in ecosystems: linking theory to observation. Trends in Ecology and Evolution 18, 648—656.
Schmitz, O.J., 2005. Scaling from plot experiments to landscapes: studying grasshoppers to inform forest ecosystem management. Oecologia 145, 225—234.
Schmitz, O.J., Kalies, E.L., Booth, M.G., 2006. Alternative dynamic regimes and trophic control of plant succession. Ecosystems 9, 659—672.
Shurin, J.B., Borer, E.T., Seabloom, E.W., Anderson, K., Blanchette, C.A., Broitman, B., Cooper, S.D., Halpern, B.S., 2002. A cross-ecosystem comparison of the strength of trophic cascades. Ecology Letters 5, 785—791.
Smith, C.J., 1943. Report of deer reduction program in Zion national Park. Unpublished report, Zion National Park Files, 12 p.
Smith, J.D., 2004. The role of riparian shrubs in preventing floodplain unraveling along the Clark Fork of the Columbia River in Deer Lodge Valley, Montana. In: Bennett, S.J., Simon, A. (Eds.), Riparian Vegetation and Fluvial Geomorpholgy, Water Science and Application, vol. 8. American Geophysical Union, Washington, DC, pp. 71—85.
Soulé, M.E., Estes, J.E., Berger, J., del Rio, C.M., 2003. Ecological effectiveness: conservation goals for interactive species. Conservation Biology 17, 1238—1250.
Soulé, M.E., Estes, J.A., Miller, B., Honnold, D.L., 2005. Strongly interacting species: conservation policy, management, and ethics. BioScience 55, 168—176.
Steens-Adams, M., 2002. Applying environmental history to restoration ecology: a case study from Zion National Park. Ecological Restoration 20, 252—261.
Stromberg, J., 1998. Dynamics of Fremont cottonwood (Populus fremontii) and saltcedar (Tamarix chinensis) populations along the San Pedro River, Arizona. Journal of Arid Environments 40, 133—135.
Terborgh, J., Estes, J.A., Paquet, P., Ralls, K., Boyd-Heigher, D., Miller, B.J., Noss, R.F., 1999. The role of top carnivores in regulating terrestrial ecosystems. In: Soulé, M., Terborgh, J. (Eds.), Continental Conservation: Scientific Foundations of Regional Reserve Networks. Island Press, Washington, DC, pp. 39—64.
Terborgh, J., Lopez, L., Nuñez, V.P., et al, 2001. Ecological meltdown in predator-free forest fragments. Science 294, 1923—1925.
Van Dyke, F.G., Brocke, R.H., Shaw, H.G., Ackerman, B.B., Hemker, T.P., Lindzey, F.G., 1986. Reactions of Mountain Lions to logging and human activities. Journal of Wildlife Management 50, 95—102.